For several years, systematic research has been carried out on the pollution of the night sky by artificial light in the city of Toruń11,36,38. The main objective is to monitor this phenomenon, including its spatial and temporal variability and the most important factors affecting it. Based on previous experience, an in-house measurement device was constructed to automate the process of data acquisition.
Genesis of the project
The first measurements pertaining to the phenomenon of night sky pollution in Toruń were made in autumn 2017, followed by regular observations using a handheld SQM photometer (Unihedron, Canada) as part of a project implemented in 2017–2018. To this end, a permanent measurement network distributed throughout the city was established, consisting of 24 locations. During a one night measurement session taking place during the astronomical night (there is no such period at the latitude of Toruń in the summer), sky brightness was measured at all sites. The results of spot monitoring were plotted using interpolation methods and visualization tools available in GIS systems11.39, which helped to determine the spatial distribution and extent of night sky light pollution. The intensity of this phenomenon at each of the surveyed points was also explored in relation to the distinguished land cover categories and types of urban development.
Repeatable measurements performed regularly over such a long period of time were characterized by significant limitations. One measurement session was very time-consuming, as it lasted about two hours, during which time all measurement locations were visited, covering a distance of almost 50 km each night by car. Despite the observance of all time frames and sticking to the plan of fieldwork, measurements were not carried out simultaneously at all the locations, which affected the results, especially during the night with changing cloud cover. Although the measurements were carried out with great consistency and care, they were performed in a spatial buffer of about 5 m, which could unintentionally slightly affect the results obtained. An additional limitation was also a one-time night measurement at one point, instead of a whole series of measurements at specific time intervals. Inaccuracies in the readings within a single session could have been caused by sudden changes in meteorological parameters. In the adopted procedure, it was not possible to carry out simultaneous measurements in identical time and weather conditions at all the locations, not to mention the involvement of the personnel in each tour of the measurement network points.
Using the experience gained and after an analysis of the identified constraints and the technical capabilities at hand, work began in 2019 on developing a network for automatic remote monitoring of light pollution of the night sky in Toruń, based on designed in-house recording devices.
Design, functional and utility features of the device
To enhance the research on light pollution in urban space, work has begun on the construction of a device that would perform automatic measurements, would be mobile, battery-powered and use long-range wireless communication. All the aforementioned features are in line with the strategy of Industry 4.0 and modern solutions proposed as part of the Smart City concept.
The concept of Industry 4.0 assumes the more and more common use of process automation as well as the processing and exchange of data with the use of new transmission technologies26. LoRaWAN is one of the solutions used for communication of Internet of Things (IoT) devices, which supports the development of Smart Cities in the Smart Environment area. As a result, the interactivity, frequency, and scope of measurements carried out in urbanized areas are increased40,41.
According to the developed project, the device was to serve as a meter of very low intensity light observed in the night sky. In this respect, it was necessary to use a sensor with technical parameters suitable for very accurate measurements of light intensity. To locally verify the weather conditions occurring during the operation of the device, it was decided to carry out additional simultaneous measurements of other environmental parameters—temperature and moisture content. The analysis of the spatial coverage of the study area indicated that 36 measurement devices should be deployed to provide full coverage of Toruń. The concept of creating an urban measurement network assumes the selection of points covering the whole city relatively evenly and representing different types of housing development and elements of land cover. It was assumed that measurements will be made only at night, between 21 pm and 6 am on the following day, at 15 min intervals, and in addition, weather conditions will be recorded twice a day.
Construction and technical parameters of the device, and selected characteristics of its components
A prototype device meeting all the predefined functionalities was constructed based on available electronic modules. The B-L072Z-LRWAN Discovery development board from STMicroelectronics42 was selected as the main electronic component providing wireless communication. This board has an integrated LoRa communication module, enabling low-power wireless messaging, and also allows the board to enter a low-power state during hibernation, and thus target long-term battery-powered operation. This module is fully programmable, which enables future expansion of the set with other functionalities. The TSL2591 light sensor from AMS, which has high sensitivity and registration accuracy, was selected as a component implementing the light intensity measurement. Its great advantage is a wide measurement range of 188 μlx to 88,000 lx, sensitivity reaching 0.000377 lx, and a wide dynamic range (WDR) of 600 M:143. The sensor used has two diodes with different spectral properties. One of them registers visible light together with infrared (in the range from 400 to 1,100 nm), while the other is responsible for the registration of infrared light (between 500 and 1,100 nm). Thanks to this solution, we can use the results in various ways. The use of the formula provided by the manufacturer allows us to obtain spectral characteristics similar to the human eye. The presence of a compensating diode makes a difference compared to the sensor used in the SQM device, so the results obtained in the measurements may be slightly different.
To measure additional environmental parameters, the X-NUCLEO-IKS01A2 development board from STMicroelectronics was used, which is connected to the STM32 microcontroller via the I2C interface44. This board enables the recording of a number of parameters, however, in the constructed device it is only responsible for reading the temperature and humidity of the environment. This results from the need to limit the size of the message packets sent, while at the same time improving the operating range and reducing the power consumption of the device.
Once all the components had been selected, tested and integrated, the process of final connection and programming was carried out. The base of the device, ie the B-L072Z-LRWAN development board was connected to the X-NUCLEO-IKS01A2 environmental sensor board, using Arduino connectors. Using standard wires, a TSL2591 light sensor was added by connecting the corresponding I2C (SCL and SDA), power supply (VIN), sensor ground (GND) pins and the X-NUCLEO-IKS01A2 board.
All components used were placed in a standard external casing with dimensions of 8.0 × 5.4 × 15.8 cm. In its lower part an opening was made for an external antenna, while in the upper part a specifically selected opening was cut out, protected with a glass pane, through which measurements are performed by the light sensor (Fig. 2).
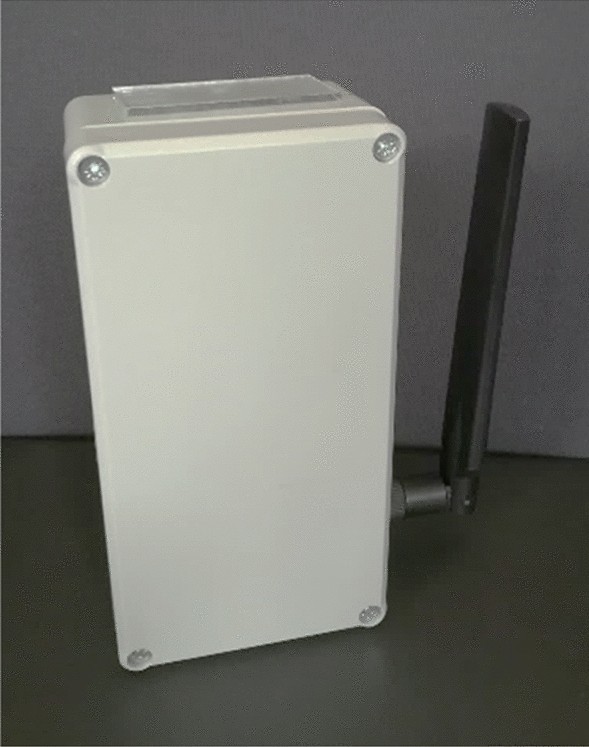
(photo by Dominika Karpińska).
Following the above steps, an automatic device was constructed to record light intensity in the lower troposphere, ie to measure the pollution of the night sky by artificial light coming from the Earth’s surface. Selected technical parameters of the device are presented in Table 1.
Flowchart of the system operation
After constructing the device and writing the control software, the construction of the entire measurement system was started. Each of the measuring instruments is ultimately connected to the communication gateway using LoRa technology. A MultiTech communication gateway with a LoRaWAN module was used as an access device. To successfully connect the gateway to the measuring device, it was necessary to configure the communication gateway software. To this end, the information about the unique device number (Dev EUI) and the application key and its number (App EUI and App Key) was used. Once the unit is configured, it is possible to send data to the communication gateway and read them using NodeRED, a programming tool where data are redirected to a selected server, which stores all measurement results. Figure 3 shows a schematic representation of the constructed measurement system.
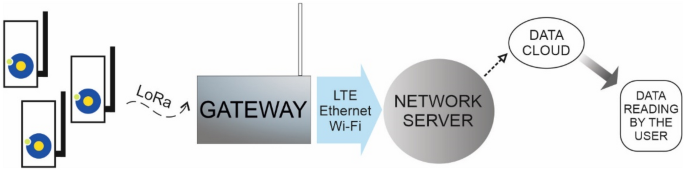
Schematic diagram of the measurement system.